Making Carbon and Climate Change Visible
Climate change is caused by greenhouse gasses that are “invisible” to the naked eye. Being aware of the accumulation of these gasses in the atmosphere can help us to understand what causes climate change. It also helps humans to understand the interconnectedness between humans and the biophysical world. Hydrogen, oxygen, carbon, and nitrogen are the most common chemical elements in the bodies of humans and all other living beings. These elements are also prominent in the atmosphere. Indeed, carbon, oxygen, and hydrogen combine themselves to form the most conspicuous greenhouse gasses: carbon dioxide (CO2) and methane (CH4). This section guides readers to learn about how these greenhouse gasses are studied in the microcosm of the peatlands and the macrocosm of the atmosphere.
Switch between the microcosm and macrocosm perspectives by clicking on the circles below.
The original virtual exhibition includes the option to switch between the microcosm and macrocosm within the individual chapters (see screenshot below).
Here we present the subchapters one after the other.
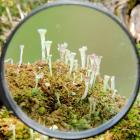
Peatlands and Global Change
At Omora Park in southern Chile as well as in other forested high-latitude regions, every fall the forest floor is carpeted by thousands of leaves, fallen from trees such as the high-deciduous beech or “lenga” (Nothofagus pumilio) and shrubs such as the wild-currant or zarzaparrilla (Ribes magellanicum). By the following spring, much of the leaves will have vanished, consumed by fungi and bacteria. These microorganisms feed on dead organic material to retrieve the energy previously locked in the cellulose that makes up the cell wall of plants. Cellulose is built from the sugar that plants produce during photosynthesis: solar energy is used to fix carbon dioxide and assemble small sugar molecules into this complex building material. Much of life on Earth relies on the sugar produced during photosynthesis to fuel all cellular processes. Indeed, when sugar is burned during respiration, energy is released, much like when wood is burned and heat is generated. When clearing large forested areas, we not only take out the trees that fix carbon into sugar, but we also release much of the carbon and energy stored in the trees. Deforestation leads to an increase in atmospheric carbon dioxide, a major greenhouse gas, which absorbs solar energy and thereby warms up the atmosphere.
Another major global carbon sink is found belowground. Organic matter that is not decomposed may accumulate sometimes over hundreds and thousands of years. In the Sphagnum-dominated peatlands, which cover over 4.4 million hectares in the subantarctic Magellanic ecoregion, the growth of bacteria and fungi is largely inhibited (Figures 1.1 and 1.2). Sphagnum is able to acidify the surrounding water to such an extent that many other organisms cannot survive. As the moss grows the older branches and parts of the stems die, and since they are not decomposed, they accumulate. Year after year, a new layer is added. Over periods of hundreds or even a few thousand years, this layer of dead organic material (i.e., peat) may reach two or three meters in thickness. As one of the most carbon-rich ecosystems, peatlands are a reservoir of energy and not surprisingly have been harvested to heat homes in large parts of the world.
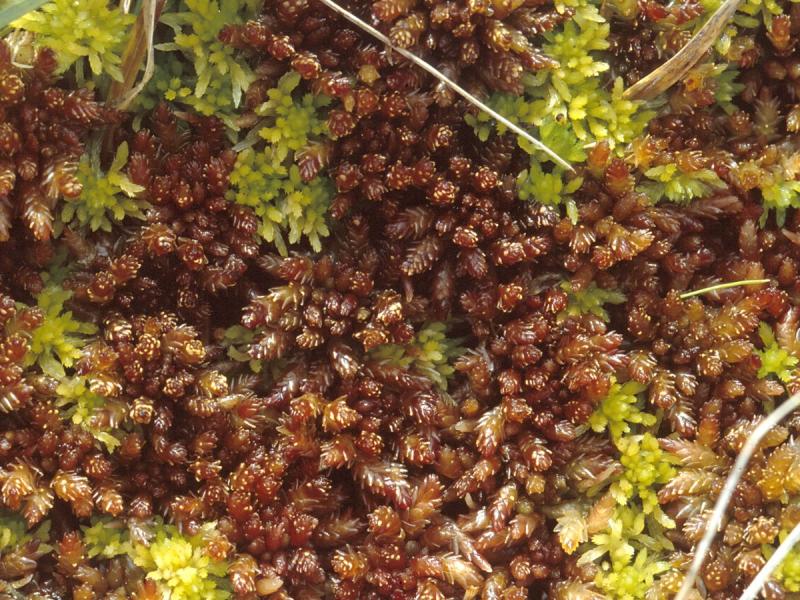
Figure 1.1. Mosses of the genus Sphagnum dominate the subantarctic peatlands forming dense and deep cushions, like those found in the lowlands of Omora Park. Sphagnum magellanicum (reddish) and S. fimbriatum (green) are the most abundant species of peat mosses in the Cape Horn Biosphere Reserve. Photograph by Paola Vezzani, n.d.
Figure 1.1. Mosses of the genus Sphagnum dominate the subantarctic peatlands forming dense and deep cushions, like those found in the lowlands of Omora Park. Sphagnum magellanicum (reddish) and S. fimbriatum (green) are the most abundant species of peat mosses in the Cape Horn Biosphere Reserve. Photograph by Paola Vezzani, n.d.
© Fundación Omora. Used by permission.
The copyright holder reserves, or holds for their own use, all the rights provided by copyright law, such as distribution, performance, and creation of derivative works.
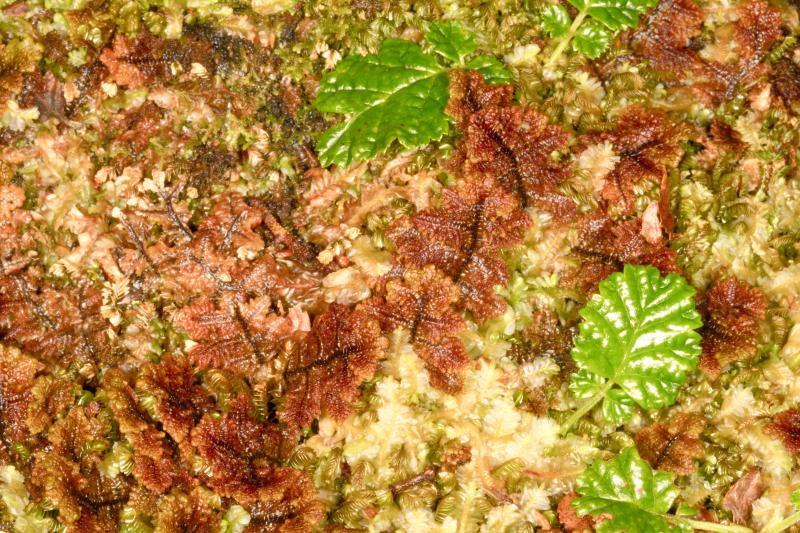
Figure 1.2. Peatlands include not only mosses of the genus Sphagnum but also a diversity of other mosses, liverworts, and small vascular plants. This picture illustrates the liverwort Gackstroemia magellanica (the dark brown reddish leafy liverwort), the moss Sphagnum magellanicum (the bright yellow), and the small vascular plant Rubus geoides (the green leaves on the right). Photograph by Adam Wilson, n.d.
Figure 1.2. Peatlands include not only mosses of the genus Sphagnum but also a diversity of other mosses, liverworts, and small vascular plants. This picture illustrates the liverwort Gackstroemia magellanica (the dark brown reddish leafy liverwort), the moss Sphagnum magellanicum (the bright yellow), and the small vascular plant Rubus geoides (the green leaves on the right). Photograph by Adam Wilson, n.d.
© Fundación Omora. Used by permission.
The copyright holder reserves, or holds for their own use, all the rights provided by copyright law, such as distribution, performance, and creation of derivative works.
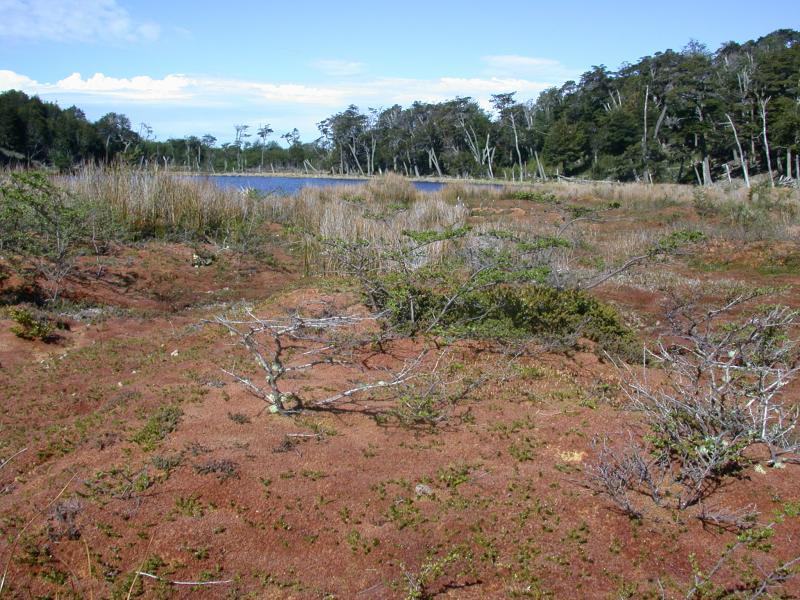
Figure 1.3. In the Cape Horn Biosphere Reserve, the subantarctic forests are embedded in a matrix of tundra. It includes vast peatlands dominated by Sphagnum magellanicum. The same species can also be encountered in the lowlands of Omora Park. The Magellanic moorland complex represents the largest wetland area at high latitude in the Southern Hemisphere. Photograph by Adam Wilson, n.d.
Figure 1.3. In the Cape Horn Biosphere Reserve, the subantarctic forests are embedded in a matrix of tundra. It includes vast peatlands dominated by Sphagnum magellanicum. The same species can also be encountered in the lowlands of Omora Park. The Magellanic moorland complex represents the largest wetland area at high latitude in the Southern Hemisphere. Photograph by Adam Wilson, n.d.
© Fundación Omora. Used by permission.
The copyright holder reserves, or holds for their own use, all the rights provided by copyright law, such as distribution, performance, and creation of derivative works.
Peatlands occur at high latitudes and altitudes, and in some areas the peat layer is several meters thick, as is the case in the lower parts of Omora Park and other sites of the Cape Horn Biosphere Reserve (CHBR) (Figure 1.3). In fact, peatlands hold more carbon, and thus energy, belowground (in peat) than do tropical rainforests aboveground (in wood).
Peat accumulates because organic material is not decomposed fast enough. The microorganisms that recycle the organic matter are inhibited by the acid water but also by the lack of oxygen. Besides acidifying the water, Sphagnum is also able to raise the water table, thereby creating an oxygen-free environment. All organisms need oxygen to burn sugar and retrieve the energy bound in cellulose, just as a candle requires oxygen to burn. If the water table drops, due to a rise in evaporation triggered by higher global temperatures or to less rainfall, microorganisms will be able to break down the peat, releasing carbon dioxide and energy into the atmosphere, amplifying the change in global climate. The amount of carbon in peatlands throughout the world is so large, and the consequences of its release so significant, that peatlands are seen as a ticking time bomb. The subantarctic peatlands form part of the Magellanic moorland complex, which is the largest wetland area at high latitude in the Southern Hemisphere, and we can keep the bomb from exploding by preserving the peatlands of Omora Park, the CHBR, and other regions of the planet.
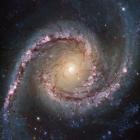
Measuring the Abundance of Greenhouse Gasses with ESO Telescopes
Earth’s atmosphere affects observations carried out by ground-based telescopes in multiple ways. One of them is the imprint of distinctive features (absorption lines) in the spectra of astronomical objects caused by the very molecules it is made of, including oxygen, nitrogen, water vapor, carbon dioxide, and methane. The shape of these features depends on temperature and pressure in the various layers of the atmosphere. To study astronomical objects, astronomers need to make sure the spectra of these objects are cleared of the features imprinted on them by Earth’s atmosphere.
To do this, astronomers used to spend precious telescope time to systematically observe “telluric standard” stars close in time and with similar coordinates as their science target. These stars are known to have no intrinsic features; hence, the ones found are identified as being caused by Earth’s atmosphere.
For the past few years, astronomers at European Southern Observatory (ESO) observatories have been able to use a different method to correct their observations thanks to an ESO software tool that creates synthetic transmission spectra of the atmosphere using the spectra of the astronomical target themselves. In addition, this method allows them to save telescope time. However, this scheme requires many calculations to determine the abundance of each molecule and may not be applicable in some cases.
In a proposed development, the number of calculations can be much reduced if, first, telluric-standard stars are regularly observed during twilight. These observations would be automatically processed by the tool only to determine the abundances of molecules other than water vapor (whose abundance can vary greatly on short timescale and therefore requires specific equipment) and would then be input into a dedicated database. Finally, the astronomer would use the tool but automatically fetch the abundances of the different gases from the database for the time of their observations instead of calculating them: in other words, the tool would automatically calculate the transmission of the atmosphere for the conditions matching the ones that took place during the observation of the science target with an accuracy of within two percent.
Hence ESO will have a database of abundances for a number of greenhouse gases determined in the heart of the Atacama Desert, which allows experts to study climate change from a unique location. The database is planned to be included in one of the Network for the Detection of Atmospheric Composition Change stations, so that it can be made available to anyone wishing to use it.
As an example, the following figure shows the evolution of the abundance of CO2 from early 2014 to March 2020, based on data obtained with the X-shooter, a multiwavelength (300–2,500 nanometer) spectrograph mounted inside one of the VLT telescopes at the ESO’s Paranal Observatory. It clearly shows that the amount of this greenhouse gas in the atmosphere above Paranal has increased by more than three percent over this period. As Paranal is located in a desert in the Southern Hemisphere, measurements show fewer seasonal variations compared to those obtained from other locations. Once in operation, the proposed scheme would regularly provide measurements of the abundance of several greenhouse gases based on data obtained with more than five instruments.
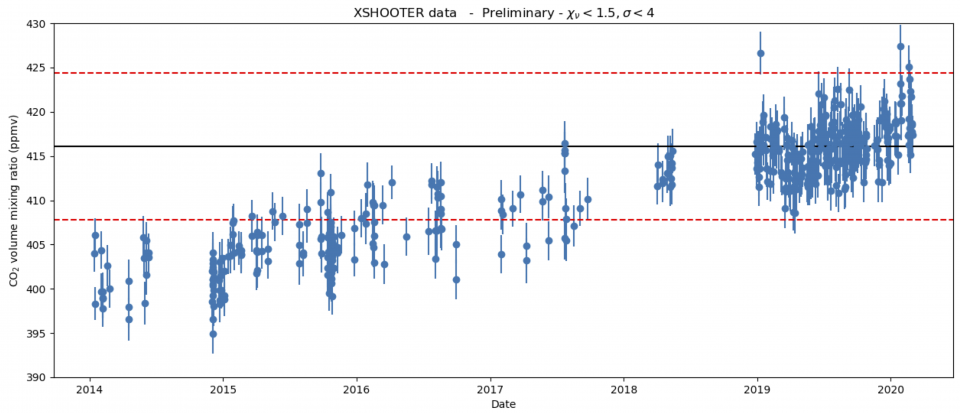
Results of a preliminary feasibility study showing the evolution of CO2 above ESO’s Paranal Observatory based on spectra obtained with the X-shooter instrument on ESO’s Very Large Telescope. The solid black line shows the mean value of the measurements obtained in 2019 while the dashed red lines correspond to +/– two percent relative to it.
Results of a preliminary feasibility study showing the evolution of CO2 above ESO’s Paranal Observatory based on spectra obtained with the X-shooter instrument on ESO’s Very Large Telescope. The solid black line shows the mean value of the measurements obtained in 2019 while the dashed red lines correspond to +/– two percent relative to it.
Courtesy of ESO/Smette.
This work is licensed under a Creative Commons Attribution 4.0 International License.
Switch between the microcosm and macrocosm perspectives by clicking on the circles above.